Keeping an eye on Cotopaxi Volcano
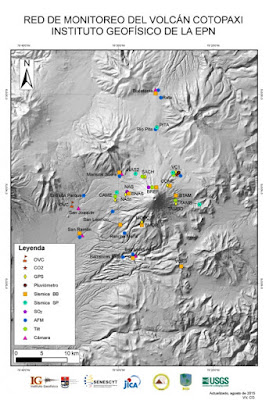
- Janine Cotopaxi volcano is Ecuador's most intensively monitored volcano. With an active past, glacier-covered summit, and surrounding population it is watched very closely by the local team of volcanologists at IGEPN . Monitoring network on and around Cotopaxi volcano that has been growing since the first seismic station installation in 1976. Courtesy of IGEPN. Cotopaxi started quietly rumbling to life again in April with an increase in seismic activity. A Seismic swarm on 14th of August preceded phreatic (water) explosions on the 15th, and now Cotopaxi is on Yellow Alert in a phase of near-continuous ash emission (for more details see the Smithsonian Reports ). The above video was posted on August 18th and shows white steam/gas plume emission and ash fall on the snowy flanks. The above video shows ash emission on the 21st of August with the ash plume that did not exceed 2 km on this day. Ashfall affected the south to west, west, and northwest of the vol